Effects of Cardiotonic Pills® on Cerebrovascular CO2 Reactivity and Erythrocyte Deformability in Normal Subjects: A Pilot Study
Article information
Abstract
Backgrounds and objectives
Cardiotonic Pills® (CP) are used for vascular diseases such as coronary diseases, atherosclerosis, and cerebral infarction. This study aimed to determine the transient effects of CP on cerebrovascular CO2 reactivity (CVR) and erythrocyte deformability in normal subjects.
Methods
This study had a crossover design and included 10 participants who were randomly allocated to 2 groups. The experimental group was given CP with water, while the control group was given only water. CVR was measured by hyperventilation-induced CVR of the middle cerebral artery (MCA) using transcranial Doppler (TCD). Erythrocyte deformability was measured using a Rheoscan-D microfluidic ektacytometer. All measurements were performed prior to and 1, 2, and 3 hours after CP or water administration. Blood pressure and heart rate were also measured before and after administration.
Results
CP significantly improved CVR 3 hours after administration in the experimental group compared to the control group (p = 0.042). The corrected blood flow velocity at partial pressure of end-tidal carbon dioxide (PETCO2) = 40mmHg (CV40) was also significantly improved 2 and 3 hours after administration in the CP group compared to the control group (p = 0.036 and p = 0.021, respectively). CP significantly improved erythrocyte deformability 3 hours after administration in the experimental group compared to the control group (p = 0.027). Mean heart rate and mean blood pressure showed no change.
Conclusions
This study demonstrated that CP increases CVR and erythrocyte deformability. These results suggested that CP improves cerebral microcirculation which provide evidence for the future use of CP for prevention of ischemic stroke and neurodegenerative diseases.
Introduction
Traditional herbal medicine is widely used in Asia along with conventional therapy to optimize the treatment of cerebrovascular disease1). According to Traditional East Asian Medicine (TEAM) principles, blood stasis is a major causative factor of cerebrovascular disease2). Blood stasis suggests a pathological state of blood stagnancy in a specific area of the body3). Recent studies demonstrated that impairments in hemorheology and microcirculation play important roles in the pathophysiology of blood stasis syndrome4,5). Therefore, improving hemorheology and microcirculation might be important when treating cerebrovascular diseases.
Cardiotonic Pills® (CP, Tasly Pharmaceuticals Inc.), a popular herbal medicine used to treat blood stasis in TEAM, is a compound drug containing Salvia miltiorrhiza (SM), Panax notoginseng (PN), and Borneolum. It is generally used in China, Korea, and Russia for the prevention and management of vascular diseases, including coronary artery disease, atherosclerosis, occlusive vasculitis, and cerebral infarction6). In previous studies, CP ameliorated microcirculatory disturbances and target organ damage resulting from ischemia and reperfusion in vivo6–8). CP also reduced platelet aggregation and enhanced erythrocyte deformability in vivo9). However, research into the effects of CP has focused on cardiovascular diseases; cerebral blood flow and microcirculation have not been extensively studied in humans.
To evaluate cerebral blood flow (CBF), cerebrovascular carbon dioxide (CO2) reactivity (CVR) is commonly measured using transcranial Doppler sonography (TCD). Cerebral vessels respond to changes in arterial carbon dioxide concentration in order to maintain constant CBF; this compensatory vasodilatory capacity of cerebral vessels is called CVR. Several studies evaluated the effect of acupuncture10–13) and herbal medicines14–16) on cerebral blood flow, based on CVR. Erythrocyte deformability is the ability of erythrocytes to fit into existing vessels, which is an important factor for microvasculature perfusion. To measure microcirculation, erythrocyte deformability is widely used. For example, a previous study revealed the effect of Hyul-Bu-Chuke-Tang on microcirculation using erythrocyte deformability17). Therefore, CVR and erythrocyte deformability are substantiated methods to evaluate CBF and microcirculation.
The purpose of this study was to evaluate the effect of CP on CBF and microcirculation by measuring the acute effects of CP on CVR using TCD to assess CBF. Erythrocyte deformability was also measured to investigate the effect of CP on microcirculation. This study was intended to extend the known cardiovascular effects of CP to cerebrovascular diseases, and ultimately provide a novel alternative for the prevention of ischemic stroke and neurodegenerative diseases.
Materials and Methods
1. Subjects
This study included 10 healthy male volunteers (mean age, 26.80 ± 0.54 years; range, 26–28 years) who were enrolled from November 2014 to December 2015. None of the subjects had a history of cerebrovascular disease, cardiac disease, diabetes mellitus, hypertension, thyroid disease, or psychiatric problems. The subjects were not allowed to drink alcohol or coffee, smoke, or take any medicine for 12 hours before study administration. Each subject was informed of the procedures before the study.
2. Materials
CP is a pharmaceutical preparation consisting of extracts from S. miltiorrhiza root (17.5 mg), P. notoginseng Radix (3.4 mg), and Borneolum (0.2 mg). CP used in this study was obtained from Tasly Pharmaceuticals Inc. (Tianjin, China).
3. Study Design
This study was designed as an open-label, crossover randomized controlled trial. The study protocol and methods were based on a previous study17). After the screening procedure, the participants were randomly allocated to 2 groups using block randomization with Excel random numbers. At the first visit (visit 1), CP (500 mg, 20 pills) with 150 mL of drinking water (group A) or only 150mL of drinking water (group B) was randomly administered to the subjects. In subsequent visit (visit 2), participants group A administered only water and vice versa. Between visit 1 and 2, a 7-day wash-out period was administered. For each participant, CVR, CBF velocity, blood pressure, heart rate, PETCO2 (partial pressure of end-tidal carbon dioxide; a measure of the amount of carbon dioxide in the exhaled air) and elongation index of erythrocyte deformability (EI) were measured at before administration (baseline), 1, 2, and 3 hours after each administration for both groups. The CP with water results of Group A and Group B were analyzed as the CP group and the only water results as the control group.
4. Measures
1) Measurement of Cerebrovascular CO2 Reactivity (CVR)
CBF velocity and CO2 reactivity of the middle cerebral artery (MCA) were measured by TCD using a Multi-Dop X4 system (Compumedics DWL, Singen, Germany) as described in previous studies10–17). CVR was measured using hyperventilation-induced CO2 reactivity of the MCA. On the first visit, the volunteer maintained a supine position and the CBF velocity of the right MCA was measured through the right temporal window. A removable bilateral probe holder (LAM-Rack; Compumedics DWL) was attached at the right temporal window to avoid shifting of the probe and to allow continuous measurement. The sample and gain values were adjusted at depths ranging from 45 to 60mm and were recorded when the waveform remained constant. After 5 minutes of stabilizing, 4 minutes of resting, and 1 minute of hyperventilation were applied to each subject while recording average CBF velocity. Average CBF velocity was measured at resting normal normocapnic status and hyperventilating hypocapnic status. All TCD waves were saved in a computer for analysis.
CO2 reactivity refers to the percent change in mean blood velocity per change in P, as calculated by the following formula. The CO2 reactivity was expressed as %/min18).
(Vrest = blood flow velocity at rest obtained during the most stable 10 seconds in the stable normocapnic condition, Vhypocapnia = blood flow velocity obtained during the most stable 10 seconds of the latter half of the 1minute hyperventilation period, = change in P from baseline to maximal hyperventilation)
The blood flow velocity is dependent on the arterial CO2 tension; thus, the corrected blood flow velocity was calculated at 40mmHg of CO2 tension (CV40, cm/s), as described previously19).
(b = CO2 reactivity, V1= velocity at P1CO2, P= end-tidal CO2 partial pressure)
To measure and control variables that may affect CBF, such as blood pressure, heart rate, and P, a Cardiocap S/5 collector (Datex-Ohmeda, Helsinki, Finland) was used in a room maintained at room temperature. Blood pressure and heart rate were measured under normocapnic conditions before hyperventilation. Blood pressure was measured using a left brachial cuff 3 times at 1-minute intervals to determine the mean blood pressure. Heart rate was continuously monitored by an oximetry apparatus positioned on the subject’s right finger. To monitor P, a nasal prong connected to the Cardiocap S/5 collector was attached in front of the subject’s nose and each subject was instructed to breath only through the nose during the examination. The Cardiocap S/5 collector software program calculated the mean heart rate and P at specific time points during the assessment. The measurements were performed before and 1, 2, and 3 hours after each administration.
2) Measurement of Erythrocyte Deformability
To measure erythrocyte deformability, a Rheoscan-D microfluidic ektacytometer (RheoMeditech, Seoul, Republic of Korea) was used. One drop of blood was obtained from each subject’s fingertip using a finger prick (Seahan Medical, Seoul, Republic of Korea). An erythrocyte suspension was prepared by mixing 6.0 μL of whole blood and 0.5 mL of a highly viscous PVP solution (31 mPa) in phosphate-buffered saline (0.14mM). A 0.5 mL aliquot of the erythrocyte suspension was placed in the test chamber of a disposable kit that included a microchannel (RheoMeditech). Differential pressure drove the erythrocyte suspension through the microchannel (0.2 × 4 × 40mm) and a laser diode was passed through the diluted mixture during the flow. The diffraction pattern of the moving erythrocytes at multiple shear stresses was projected onto a screen and the images were captured by a CCD-video camera every 0.5 seconds. The images were analyzed using an ellipse-flitting computer program. The average shear stress ranged from 0 to 30 Pa. The EI was defined as (L − W)/(L + W), where L is the major axis and W is the minor axis of the erythrocyte ellipse at the shear stress of 3 Pa20). The microchannel was discarded after each measurement.
5. Ethical approval
This study has passed the ethical review by the Institutional Review Board of Kyung Hee University Korean Medicine Hospital (KOMCIRB-150720-HR-024, approved on 1 October 2015) and strictly followed “The Helsinki Declaration” by the World Medical Association. Participants signed written informed consent after receiving a full explanation of the study from the researcher, and participants’ information was protected.
6. Statistical analysis
Statistical analysis was performed with the Statistical Package for the Social Sciences version 12.0 for Windows (SPSS, Chicago, IL). Repeated-measures ANOVA was used to compare differences in all variable means across time by group and time. An independent t-test was used to compare the differences in value changes (follow-up minus baseline) between the 2 groups. All values were corrected using the Bonferroni correction. Data are summarized as mean ± standard deviation and a p < 0.05 was considered significant.
Results
1. Clinical Baseline Characteristics
Ten normal male subjects were randomly allocated to 2 groups. One participant from group A dropped out during the second visit with water administration after declining to continue. Therefore, 10 subjects completed the CP group evaluations and 9 subjects completed the control group evaluations. This procedure is summarized in Figure 1. The baseline characteristics of the participants are summarized in Table 1. There were no significant differences in these characteristics between the 2 groups.
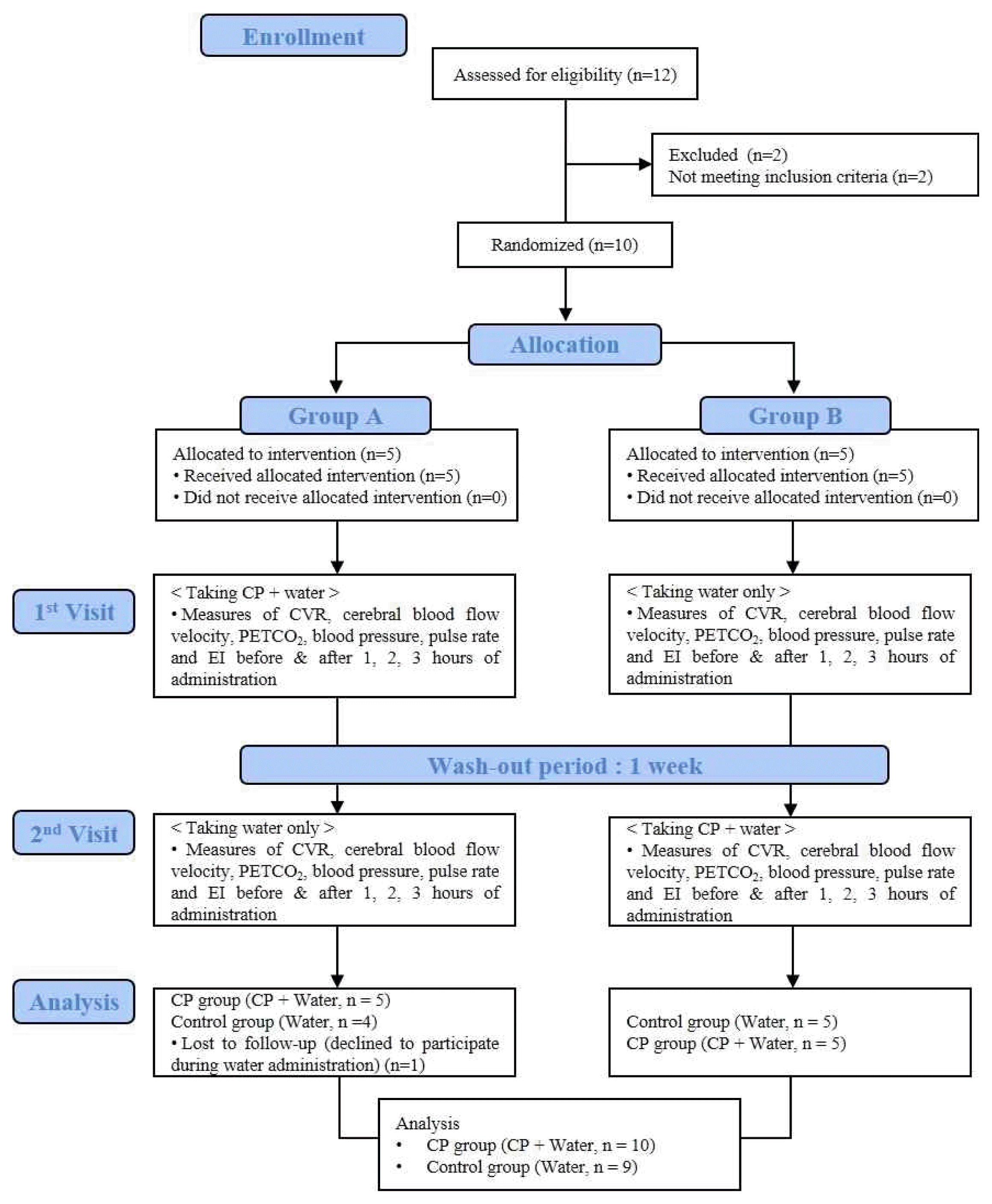
Flow chart of the study procedure
CP, Cardiotonic Pills; CVR, Cerebrovascular CO2 Reactivity; PETCO2, Partial Pressure of End-Tidal Carbon Dioxide; EI, Elongation Index of Erythrocyte Deformability.
2. Cerebrovascular CO2 Reactivity (CVR)
CVR exhibited an increasing tendency, but no statistical significance (interaction effect: F = 2.012, p = 0.124). CVR was increased at 3 hours compared to baseline in the CP group (1.88 ± 0.26 versus 2.28 ± 0.32, p = 0.042), whereas there was no significant change in the control group (Table 2). When comparing the percent change in CVR between the 2 groups, we found that CP improved CVR significantly 3 hours after administration compared to the control group (24.47 ± 8.50% versus −3.25 ± 4.72%, p = 0.042) (Figure 2).

Percent changes in CVR measures at baseline and 1, 2, and 3 hours after CP (n = 10) and Control (n = 9)
The lines represent the mean± standard deviation. CP, Cardiotonic Pills; h, hour; CVR, Cerebrovascular CO2 Reactivity. *, p<0.05 (statistically significant compared with control group, with independent t-test).
3. Corrected Blood Flow Velocity at PETCO2 = 40mmHg (CV40)
Subjects in the CP group showed a significant improvement in CV40 (interaction effect: F = 3.873, p = 0.027), whereas the control group showed a decreasing tendency with no statistical significance (Table 3). When comparing the percent change in CV40 between the 2 groups, we found that CP improved CV40 significantly 2 and 3 hours after administration compared to the control group (5.20 ± 2.76% versus −5.56 ± 2.65%, p = 0.036; 9.60 ± 4.94% versus −8.53 ± 2.87%, p = 0.021, respectively) (Figure 3).
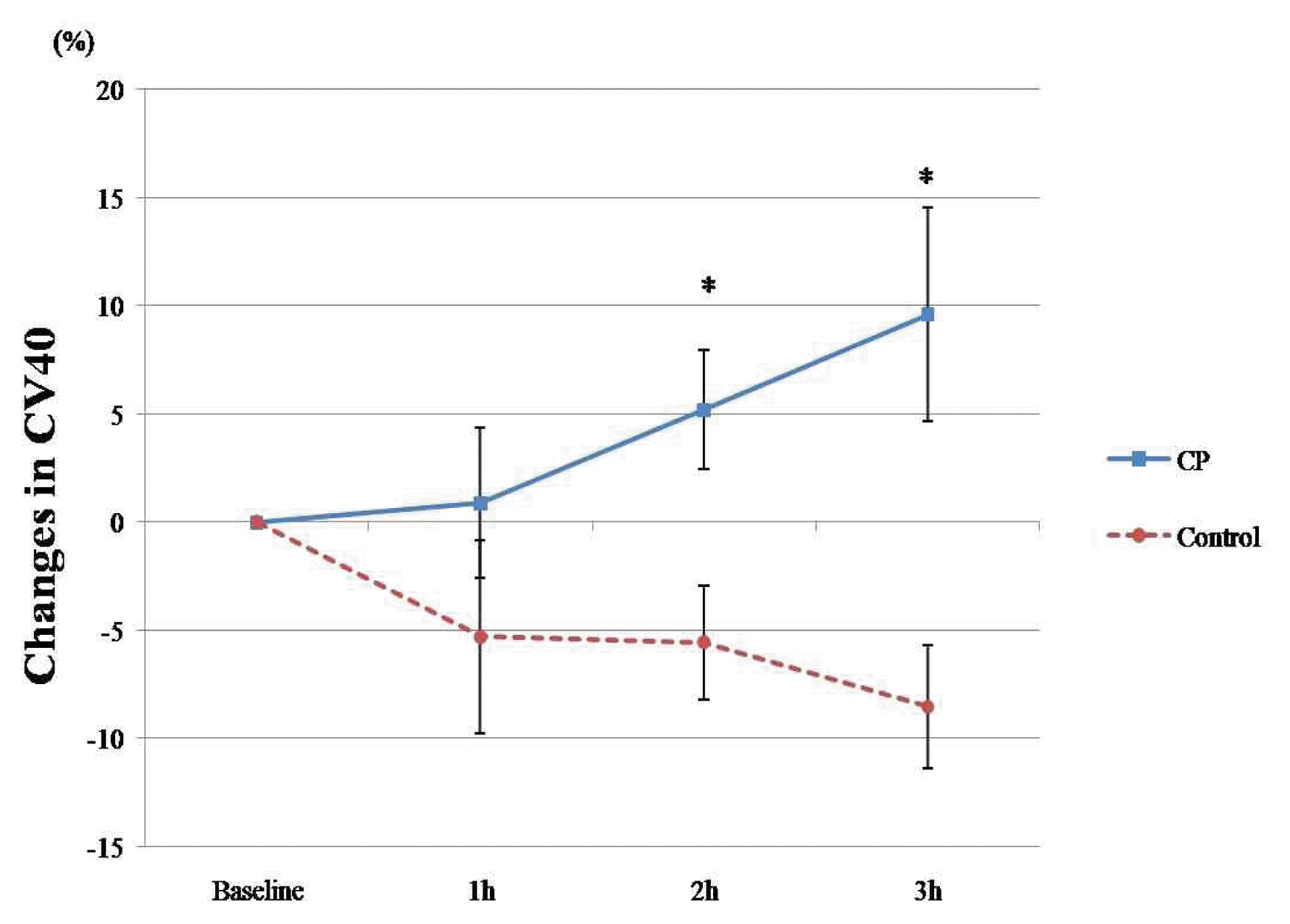
Percent changes in CV40 measures at baseline and 1, 2, and 3 hours after CP (n = 10) and Control (n = 9)
The lines represent the mean± standard deviation. CP, Cardiotonic Pills; h, hour; CV40, Corrected Blood Flow Velocity at PETCO2 = 40mmHg.
*, p<0.05 (statistically significant compared with control group, with independent t-test).
4. The Elongation Index of Erythrocyte Deformability (EI)
In the CP group, the EI showed an increasing tendency, but no statistical significance (interaction effect: F = 2.675, p = 0.057). The EI at 3 hours was significantly greater than baseline in the CP group (0.311 ± 0.004 versus 0.301 ± 0.004, p = 0.012). The EI in the control group was not altered (Table 4). When comparing the percent change in EI between the 2 groups, CP administration significantly improved EI compared to the control group 3 hours after administration (3.49 ± 1.19% versus −0.74 ± 1.18%, p = 0.027) (Figure 4).
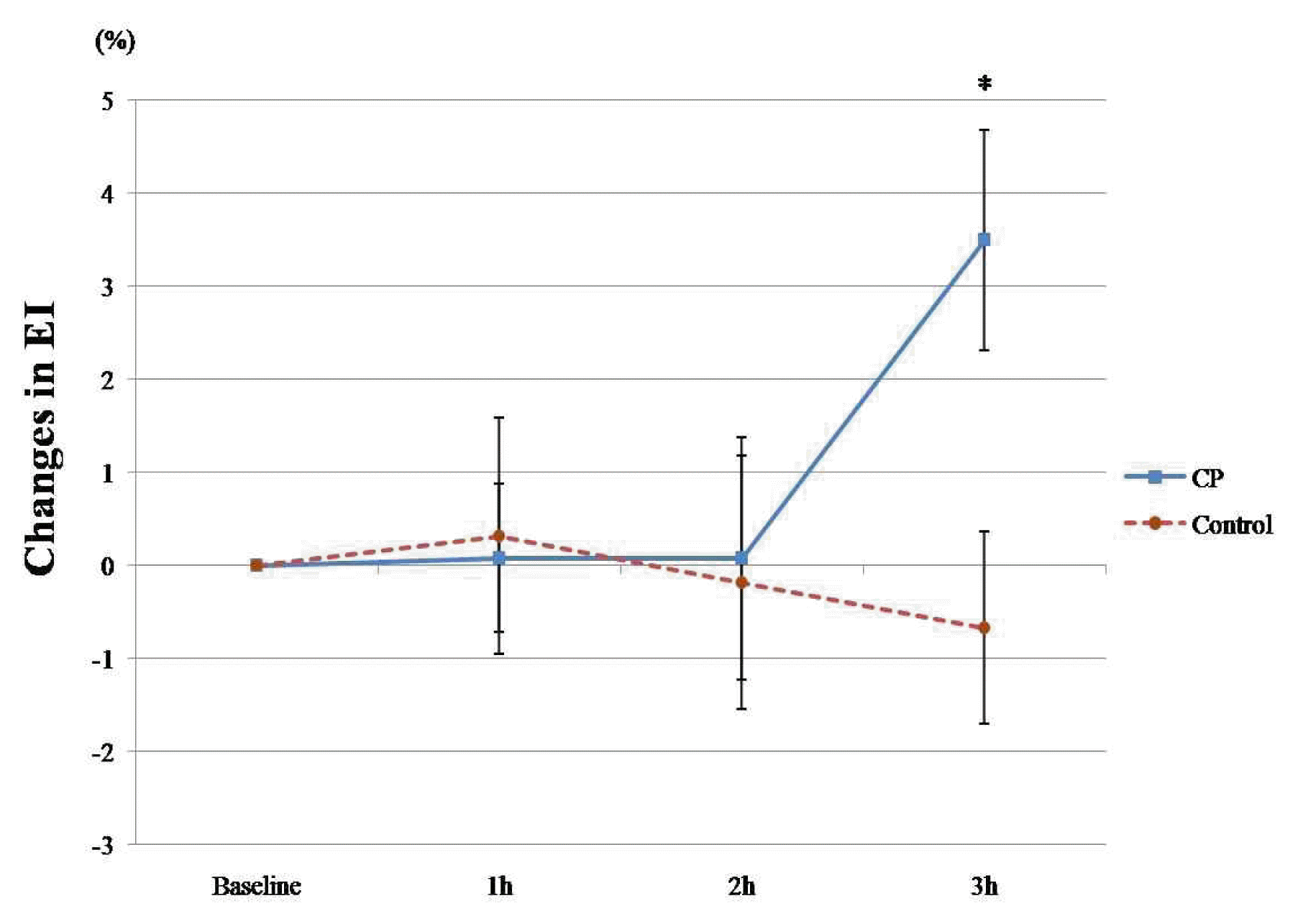
Percent changes in EI measures at baseline and 1, 2, and 3 hours after CP (n = 10) and Control (n = 9)
The lines represent the mean± standard deviation. CP, Cardiotonic Pills; h, hour; EI, Elongation Index of Erythrocyte Deformability. *, p<0.05 (statistically significant compared with control group, with independent t-test).
5. Average Blood Pressure and Average Heart Rate
Average blood pressure did not change from baseline values during the 3 hours TCD procedure in either group (Table 5). Average heart rate showed a decreasing tendency in both groups from baseline values, but there was no significant difference between the groups (Table 6).
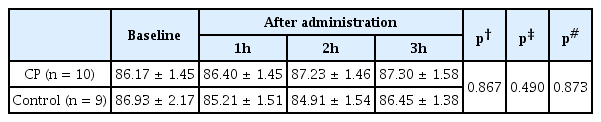
Average Blood Pressure Measures at Baseline and 1, 2, and 3 Hours after Either CP or Water Administration
6. Safety and tolerability
No significant adverse events were reported during the study.
Discussion
In this study, it was founded that CP treatment increased CVR in the MCA and improved erythrocyte deformability in young healthy subjects. These results suggested that CP exhibited acute effects on cerebral microcirculation and that CP decreased blood flow resistance in distal vessels by improving erythrocyte deformability, which contributed to the increase in CVR.
A significant increase in CVR after 3 hours and CV40 after 2 and 3 hours was confirmed after CP administration compared to baseline and the control group, whereas the mean blood pressure and heart rate did not change significantly. This result suggested that CP increased regional resting cerebral blood flow. Considering patients with stroke risk factors and stroke survivors show impaired CO2 reactivity21,22) and reduced CVR implies a greater risk of ipsilateral stroke and transient ischemic attack23), the results of this study indicate that CP can be used to improve cerebral hemodynamics. These results correspond with those of previous studies indicating that CP affects microcirculatory regulation7,24).
This study indirectly evaluated changes in CBF by assessing CVR and CBF velocity using TCD. The measurement of CBF velocity corresponds to the assessment of CBF, which is supported by previous studies. A previous study used TCD to show that, under the hypothesis that vessel diameter did not change, the MCA blood flow velocity-PETCO2 response curve was similar to the CBF-PCO2 response curve25). Therefore, a change in CBF velocity indicated a change in CBF. Also, blood flow velocity in defined cerebral arteries is significantly positively correlated with the corresponding regional CBF26) and MCA blood flow velocity correlated with carotid artery blood flow27). Therefore, it is possible that the increased CBF resulted from CP administration.
This study demonstrated that CP reduced microcirculatory resistance by improving erythrocyte deformability. Erythrocyte deformability is the ability of erythrocytes to fit into existing vessels and is an important factor in microvasculature perfusion. Erythrocytes must change shape to pass through microvessels, such as capillaries, which maintain a diameter smaller than erythrocytes28). Diminished erythrocyte deformability increases microcirculatory resistance29), which can cause various diseases, including cerebrovascular diseases. In contrast, an increase in erythrocyte deformability eases passage through capillaries, which increases the number of perfused capillaries in the vascular bed (i.e., capillary recruitment)30). The results of this study are supported by previous studies that showed that SM and PN, components of CP, increased erythrocyte deformability, inhibited platelet aggregation, lowered blood viscosity, improved peripheral microcirculation, and removed blood stasis8,9,31,32).
CP is an herbal preparation consisting of SM, PN, and Borneolum. TEAM suggests that CP impacts blood stasis syndrome, which is a pathological state of blood stagnancy in a specific area of the body3). It is widely used for the treatment, prevention, and management of vascular diseases, including coronary artery disease, atherosclerosis, occlusive vasculitis, and cerebral infarction6). Impairments in hemorheology and microcirculation play important roles in the pathophysiology of blood stasis syndrome, which is consistent with TEAM theory4,5). Recent research demonstrated that SM increased myocardial capillary density and reduced infarct size and peroxide production after myocardial infarction in rats33,34). In addition, it was previously demonstrated that the major components of SM aqueous extract improved I/R induced microcirculatory disturbance and organ injury function by reducing peroxide production, leukocyte adhesion, and albumin leakage in rat mesentery following I/R8,35). PN, another component of CP, protected the brain and liver from ischemic injury; this effect is ascribed to its inhibition of inflammation through attenuation of tumor necrosis factor (TNF-α) production and Bcl-2, caspase-1, and caspase-3 expression36). Pretreatment with ginsenoside Rb1, a major ingredient of PN, attenuated myocardial I/R injury, an effect partly mediated by activating the phosphatidylinositol 3-kinase/AKT-dependent pathway. Similar to the above herb complexes, CP diminished microcirculatory disturbances and oxidative stress in rats with myocardial ischemia/reperfusion injury via the repression of nicotinamide adenosine dinucleotide phosphate (NADPH) oxidase37). Furthermore, it has been suggested that CP might suppress thrombus development from the interaction of platelets with endothelium38). Taken together, these findings indicate that CP induced an improvement in regional blood flow as a result of reduced cerebral microcirculatory resistance.
In this study, CP improved CBF due to several possible underlying mechanisms. The first was related to blood pressure and heart rate. This study indicated that blood pressure and heart rate did not change after CP administration, which meant the increase in CBF was not caused by an increase in blood pressure or heart rate. The second was related to the activity of vascular endothelium-derived relaxing factors (VEDRF). VEDRFs control cerebral blood flow by altering vessel diameter. Among VEDRFs, nitric oxide (NO) is thought to improve vasodilation, increase blood flow, decrease vascular resistance, inhibit platelet aggregation, enhance antioxidant activity, and protect vascular endothelial cells39–41). Basal NO is continuously derived from cerebrovascular endothelium and has an intensive effect on the regulation of CBF and vascular tone42). L-arginine, which is known to increase vascular endothelial cell NO levels, improved CBF velocity in normal subjects43). In addition, externally generated NO increased erythrocyte deformability in healthy male volunteer blood samples44). It may also have ameliorated erythrocyte deformability, which would have led to increased CBF. The third was an antioxidant-induced erythrocyte deformability effect. The antioxidant defense mechanism determined the grade of red blood cell (RBC) structural rigidity45) because the RBC membrane is rich in polyunsaturated fatty acids, which created susceptibility to oxidative damage46). CP has an antioxidant effect in that it acts on multiple targets, including inhibition of NADPH oxidase, elevation of GSH, and reaction with ROS via phenolic hydroxyl groups38). However, in this study, the measurement of erythrocyte deformability was done at a peripheral artery; therefore, the connection between improved erythrocyte deformability induced by the antioxidant effect of CP and CBF is not clear.
The significance of this study is as follows: First, it expands the scope of CP disease to include cerebrovascular disease, which until now was mainly limited to cardiovascular disease. Until now, the effect of CP on cardiovascular disease has been verified through many clinical trials, but to our knowledge, this study is the first study to verify the applicability of CP for cerebrovascular disease in humans. Therefore, it is meaningful that it presented a milestone for clinical trials for cerebrovascular disease of CP in the future. Second, it provides a novel alternative to primary prevention of ischemic stroke. Aspirin is currently recommended in guidelines for the primary prevention of ischemic stroke, especially for those at high risk for cardiovascular disease47). However, the effectiveness of aspirin for primary prevention of ischemic stroke remains controversial48), and Asians in particular have been shown to have a higher incidence of aspirin-induced hemorrhagic stroke than Westerners49,50). Therefore, there is a need for a more effective and safer alternative to aspirin as a primary prevention agent of ischemic stroke for people at high risk of cerebrovascular disease. The CRF improvement in normal subjects in this study suggests that CP has potential as a primary prevention agent for ischemic stroke. Finally, this study presents a potential prevention agent for neurodegenerative diseases. Since an adequate blood supply plays a key role in normal brain functioning51), CBF and CVR impairments have been related to neurodegenerative diseases such as Alzheimer’s disease, vascular dementia52), and Parkinson’s disease53). Considering that there are no effective preventive measures for neurodegenerative diseases, the results of this study confirm its potential as a preventive agent for neurodegenerative diseases. Future clinical studies will be needed to validate the findings of this study.
The present study has a few limitations. No placebo drug was used for the control group because of the difficulty of making a placebo with the same flavor and scent as CP. Another limitation was the small sample size. Finally, the quality of the research evidence is compromised by the fact that the experimental and control groups are the same group with a wash out period, and the researchers and subjects are not blinded. As this is a pilot study to explore the potential application of CP in cerebrovascular disease, further blind randomized controlled trials with larger subject groups and placebo in stroke patients may be required to confirm the present findings.
Conclusions
This study assessed the short-term effects of a single administration of Cardiotonic Pills® CVR and erythrocyte deformability in normal subjects. CVR was significantly increased 3 hours after CP administration compared to control group and CV40 showed significant improvement in the CP group after 2 and 3 hours compared to the control group. Erythrocyte deformability was markedly increased 3 hours after CP administration compared to the control group. Mean blood pressure and heart rate showed no significant change. These findings indicate that CP could improve acute cerebrovascular blood flow in normal subjects, which may warrant the expanded application of CP for cerebrovascular diseases. Ultimately, this study provides evidence for the future application of CP as primary prevention of ischemic stroke and prevention of neurodegenerative diseases.
Acknowledgements
The authors would like to thank all participants in this study, all the staffs for coordinating the work and all investigators for data collection and analysis.
Notes
Funding
This research was supported by a grant of the Korea Health Technology R&D Project through the Korea Health Industry Development Institute (KHDI), funded by the Ministry of Health & Welfare, Republic of Korea (grant number: HF20C0113).